Algae Gravitational Sinking Velocities and Carbon Sequestration Research
Phase 1: Introduction
While surfing online I found an interesting article about algae and their ability to sequester carbon in the ocean. This topic deeply resonated with me because I am concerned about the negative consequences of climate change being biodiversity loss, wildfires, and glacial melting. I learned that algae are photosynthetic organisms that play an important role in marine food webs. Motile species of unicellular algae rise and sink to access nutrients at different depths in the ocean. This includes rising to the ocean surface for sunlight and atmospheric carbon dioxide that has diffused into the ocean to drive photosynthesis and consequently produce energy. When algae die and sink, the carbon they've absorbed is then sequestered deep on the ocean floor, effectively removing it from the atmosphere for long periods. This natural process contributes to reducing atmospheric carbon levels, aiding in climate regulation because carbon dioxide is a greenhouse gas that traps heat in the atmosphere having catastrophic effects on the environment. Currently, I am working alongside PhD Teemu Miettinen to better understand the mechanisms by which algae modulate their sinking velocities.
Phase 2: How Do Algae Sink?
Inorganic and organic nutrients are found deep in the ocean while sunlight is more direct at the surface. Thus, algae must adjust their vertical motion in the water so that they can thrive. Algae's gravitational sinking velocity is dependent on density, mass, and drag force from seawater and can be derived using Stokes' Law. To obtain the density, the buoyant mass and volume of the algae must be found. Buoyant mass is achieved using a suspended microchannel resonator, or SMR. In the SMR, cells flow through a microchannel inside a vibrating cantilever. As the cells flow through the microchannel the minute vibrations of the cantilever are proportional to the buoyant mass. For the volume, cells pass through a fluorescence detection area and the fluorescence signal decreases in proportion to the volume of an algae cell traveling through. Using the formula mass/volume, the density of singular algae can be found.
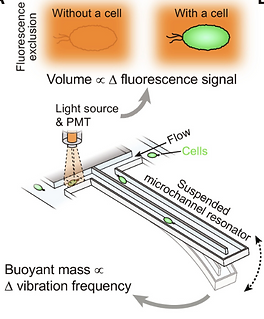
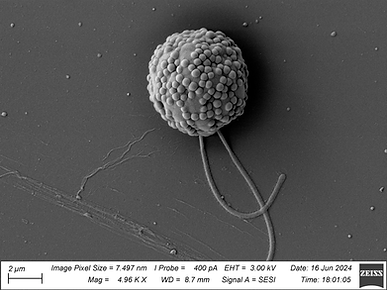
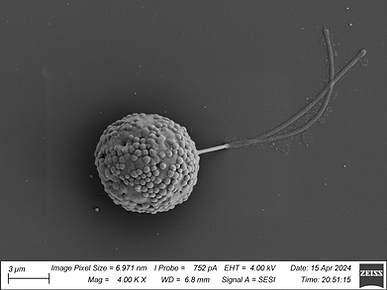
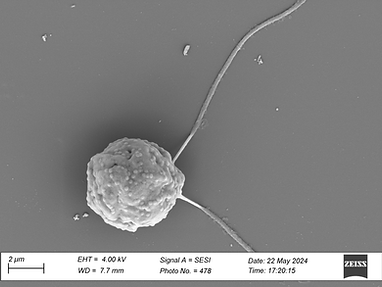

Phase 3: Lipid Quantifications
When algae cells are at the surface of the water they need nutrients which requires them to sink back to the bottom of the ocean. Algae can do this by changing their mass or volume. A volume change would be marked by talking in more water, however, there are many aspects to how green algae can change their mass in nutrient-deficient conditions. One change in composition is an increase in lipid production. Species of algae were tested in high nutrient (adequate), low nutrient, no phosphorus, and no nitrogen conditions. In SEM as well as TEM microscopic images of these algae there appears to be an increase in the size of lipid droplets. I wrote a MATLAB script to aid in quantified over 3,000 lipid droplets from algae images. The results reveal that lipid droplets are about 2.5 times larger in low-nutrient conditions than in high, indicating that this is a mechanism by which algae cells increase their mass to adjust their position in the ocean.


Phase 4: Starch Quantifications
Another way in which algae can store carbon is in the form of glucose which can then be processed by cells into starch. We modified our MATLAB codebase to determine the starch composition of individual algae cells based on TEM images of their cross-sections. Algal species of Chlorodendrophyceae, with ID CCMP908, and Dunaliella tertiolecta, with ID CCMP362 were investigated. These algae cells had been introduced to, once again, high nutrient, low nutrient, and no nitrogen conditions before microscopy processes. Our code allowed the user to outline the cell and then individual starch granules inside the cell for over 500 algae images. The MATLAB script determined the percent starch in each cell, the area of the cross-section, the number of starch granules, and the area of starch granules per cell.


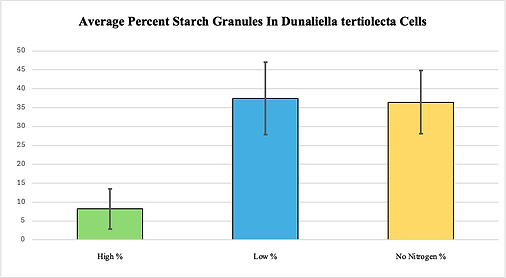
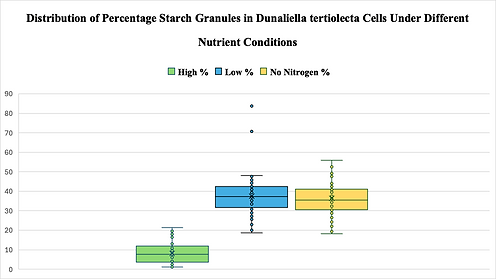
Phase 5: Starch Data Analysis
After running extensive statistical testing, conclusions were drawn. For the 908 species, the percentage of granules in cells under low-nutrient and no-nitrogen conditions is about six times higher than in cells under high-nutrient conditions. The t-tests confirm that the differences in the percentage of granules between high-nutrient and low-nutrient cells, as well as between high-nutrient and no-nitrogen cells, are statistically significant. Therefore, we can reject the null hypothesis that the means of these populations are the same. Additionally, on average, cells in low-nutrient and no-nitrogen conditions have twice the number of starch granules as those in high-nutrient conditions, although this comparison may be influenced by differences in cell size. These results are similar for the 362 species, except that the percentage of granules in low-nutrient and no-nitrogen cells is approximately 4.6 times higher than in high-nutrient cells. This suggests that starch granules could play a role in increasing the density of algae cells in both the 362 and 908 species. However, for the 362 species, the increase in granules does not appear to affect cell volume, as t-tests show no statistically significant differences in cell area across conditions. If the area of the cell cross-section increases, the volume of the cell would also increase. This is because if we assume that cells are spherical and cross-sections are circles, an increase in radius would lead to an increase in volume. For the 908 species, cell area is significantly different between high-nutrient and low-nutrient or no-nitrogen conditions, indicating that an increase in starch granules may also increase cell volume, potentially enhancing sinking velocity. Overall, carbon accumulation is likely a mechanism used by various species of algae to increase their sinking velocity because an increase in starch would lead to an increase in mass. Carbon sequestration would also be more efficient when algae are under low-nutrient or no-nitrogen conditions because they accumulate more starch. This information is useful as photobioreactors gain popularity because algal nutrient conditions could be manipulated in enclosed vessels to optimize carbon sequestration.